Earthquake
An earthquake (also known as a quake, tremor or temblor) is the result of a sudden release of energy in the Earth's crust that creates seismic waves.

Tsunami
A tsunami also called a tsunami wave train, and at one time incorrectly referred to as a tidal wave, is a series of water waves caused by the displacement of a large volume of a body of water, usually an ocean, though it can occur in large lakes.

Tornado
A tornado (often referred to as a twister or, erroneously, a cyclone) is a violent, dangerous, rotating column of air that is in contact with both the surface of the earth and a cumulonimbus cloud or, in rare cases, the base of a cumulus cloud.

Floods
A flood is an overflow of an expanse of water that submerges land. The EU Floods directive defines a flood as a temporary covering by water of land not normally covered by water

Volcanic Eruptions
Volcanoes can cause widespread destruction and consequent disaster through several ways. The effects include the volcanic eruption itself that may cause harm following the explosion of the volcano or the fall of rock.

Tsunami Warnings and Predictions
7:48 AM
Posted by Disaster

Drawbacks can serve as a brief warning. People who observe drawback (many survivors report an accompanying sucking sound), can survive only if they immediately run for high ground or seek the upper floors of nearby buildings. In 2004, ten-year old Tilly Smith of Surrey, England, was on Maikhao beach in Phuket, Thailand with her parents and sister, and having learned about tsunamis recently in school, told her family that a tsunami might be imminent. Her parents warned others minutes before the wave arrived, saving dozens of lives. She credited her geography teacher, Andrew Kearney.
In the 2004 Indian Ocean tsunami drawback was not reported on the African coast or any other eastern coasts it reached. This was because the wave moved downwards on the eastern side of the fault line and upwards on the western side. The western pulse hit coastal Africa and other western areas.
A tsunami cannot be precisely predicted, even if the magnitude and location of an earthquake is known. Geologists, oceanographers, and seismologists analyse each earthquake and based on many factors may or may not issue a tsunami warning. However, there are some warning signs of an impending tsunami, and automated systems can provide warnings immediately after an earthquake in time to save lives. One of the most successful systems uses bottom pressure sensors that are attached to buoys. The sensors constantly monitor the pressure of the overlying water column. This is deduced through the calculation:
where
P = the overlying pressure in newtons per metre square,
ρ = the density of the seawater= 1.1 x 103 kg/m3,
g = the acceleration due to gravity= 9.8 m/s2 and
h = the height of the water column in metres.
Hence for a water column of 5,000 m depth the overlying pressure is equal to
or about 5500 tonnes-force per square metre.
Regions with a high tsunami risk typically use tsunami warning systems to warn the population before the wave reaches land. On the west coast of the United States, which is prone to Pacific Ocean tsunami, warning signs indicate evacuation routes. In Japan, the community is well-educated about earthquakes and tsunamis, and along the Japanese shorelines the tsunami warning signs are reminders of the natural hazards together with a network of warning sirens, typically at the top of the cliff of surroundings hills.
The Pacific Tsunami Warning System is based in Honolulu, Hawaiʻi. It monitors Pacific Ocean seismic activity. A sufficiently large earthquake magnitude and other information triggers a tsunami warning. While the subduction zones around the Pacific are seismically active, not all earthquakes generate tsunami. Computers assist in analysing the tsunami risk of every earthquake that occurs in the Pacific Ocean and the adjoining land masses.
As a direct result of the Indian Ocean tsunami, a re-appraisal of the tsunami threat for all coastal areas is being undertaken by national governments and the United Nations Disaster Mitigation Committee. A tsunami warning system is being installed in the Indian Ocean.Computer models can predict tsunami arrival, usually within minutes of the arrival time. Bottom pressure sensors relay information in real time. Based on these pressure readings and other seismic information and the seafloor's shape (bathymetry) and coastal topography, the models estimate the amplitude and surge height of the approaching tsunami. All Pacific Rim countries collaborate in the Tsunami Warning System and most regularly practice evacuation and other procedures. In Japan, such preparation is mandatory for government, local authorities, emergency services and the population.
Some zoologists hypothesise that some animal species have an ability to sense subsonic Rayleigh waves from an earthquake or a tsunami. If correct, monitoring their behavior could provide advance warning of earthquakes, tsunami etc. However, the evidence is controversial and is not widely accepted. There are unsubstantiated claims about the Lisbon quake that some animals escaped to higher ground, while many other animals in the same areas drowned. The phenomenon was also noted by media sources in Sri Lanka in the 2004 Indian Ocean earthquake. It is possible that certain animals (e.g., elephants) may have heard the sounds of the tsunami as it approached the coast. The elephants' reaction was to move away from the approaching noise. By contrast, some humans went to the shore to investigate and many drowned as a result.
Along the United States west coast, in addition to sirens, warnings are sent on television & radio via the National Weather Service, using the Emergency Alert System.
Tsunami Scales of Intensity and Magnitude
7:45 AM
Posted by Disaster
As with earthquakes, several attempts have been made to set up scales of tsunami intensity or magnitude to allow comparison between different events.
Intensity scales
The first scales used routinely to measure the intensity of tsunami were the Sieberg-Ambraseys scale, used in the Mediterranean Sea and the Imamura-Iida intensity scale, used in the Pacific Ocean. The latter scale was modified by Soloviev, who calculated the Tsunami intensity I according to the formula
where Hav is the average wave height along the nearest coast. This scale, known as the Soloviev-Imamura tsunami intensity scale, is used in the global tsunami catalogues compiled by the NGDC/NOAA and the Novosibirsk Tsunami Laboratory as the main parameter for the size of the tsunami.
Magnitude scales
The first scale that genuinely calculated a magnitude for a tsunami, rather than an intensity at a particular location was the ML scale proposed by Murty & Loomis based on the potential energy. Difficulties in calculating the potential energy of the tsunami mean that this scale is rarely used. Abe introduced the tsunami magnitude scale Mt, calculated from,
Characteristics of Tsunami
7:43 AM
Posted by Disaster
Tsunamis cause damage by two mechanisms: the smashing force of a wall of water travelling at high speed, and the destructive power of a large volume of water draining off the land and carrying all with it, even if the wave did not look large.
While everyday wind waves have a wavelength (from crest to crest) of about 100 metres (330 ft) and a height of roughly 2 metres (6.6 ft), a tsunami in the deep ocean has a wavelength of about 200 kilometres (120 mi). Such a wave travels at well over 800 kilometres per hour (500 mph), but owing to the enormous wavelength the wave oscillation at any given point takes 20 or 30 minutes to complete a cycle and has an amplitude of only about 1 metre (3.3 ft). This makes tsunamis difficult to detect over deep water. Ships rarely notice their passage.
As the tsunami approaches the coast and the waters become shallow, wave shoaling compresses the wave and its velocity slows below 80 kilometres per hour (50 mph). Its wavelength diminishes to less than 20 kilometres (12 mi) and its amplitude grows enormously. Since the wave still has the same very long period, the tsunami may take minutes to reach full height. Except for the very largest tsunamis, the approaching wave does not break, but rather appears like a fast-moving tidal bore. Open bays and coastlines adjacent to very deep water may shape the tsunami further into a step-like wave with a steep-breaking front.
When the tsunami's wave peak reaches the shore, the resulting temporary rise in sea level is termed run up. Run up is measured in metres above a reference sea level. A large tsunami may feature multiple waves arriving over a period of hours, with significant time between the wave crests. The first wave to reach the shore may not have the highest run up.
About 80% of tsunamis occur in the Pacific Ocean, but they are possible wherever there are large bodies of water, including lakes. They are caused by earthquakes, landslides, volcanic explosions, and bolides.
Tsunami Cause or Generation Mechanisms
7:34 AM
Posted by Disaster

The principal generation mechanism or cause of a tsunami is the displacement of a substantial volume of water or perturbation of the sea. This displacement of water is usually attributed to either earthquakes, landslides, volcanic eruptions,glacier calvings or more rarely by meteorites and nuclear tests. The waves formed in this way are then sustained by gravity. Tides do not play any part in the generation of tsunamis.
Tsunami generated by seismicity
Tsunami can be generated when the sea floor abruptly deforms and vertically displaces the overlying water. Tectonic earthquakes are a particular kind of earthquake that are associated with the Earth's crustal deformation; when these earthquakes occur beneath the sea, the water above the deformed area is displaced from its equilibrium position. More specifically, a tsunami can be generated when thrust faults associated with convergent or destructive plate boundaries move abruptly, resulting in water displacement, owing to the vertical component of movement involved. Movement on normal faults will also cause displacement of the seabed, but the size of the largest of such events is normally too small to give rise to a significant tsunami.
Tsunamis have a small amplitude (wave height) offshore, and a very long wavelength (often hundreds of kilometers long, whereas normal ocean waves have a wavelength of only 30 or 40 metres), which is why they generally pass unnoticed at sea, forming only a slight swell usually about 300 millimetres (12 in) above the normal sea surface. They grow in height when they reach shallower water, in a wave shoaling process described below. A tsunami can occur in any tidal state and even at low tide can still inundate coastal areas.
On April 1, 1946, a magnitude-7.8 (Richter Scale) earthquake occurred near the Aleutian Islands, Alaska. It generated a tsunami which inundated Hilo on the island of Hawai'i with a 14 metres (46 ft) high surge. The area where the earthquake occurred is where the Pacific Ocean floor is subducting (or being pushed downwards) under Alaska.
Examples of tsunami originating at locations away from convergent boundaries include Storegga about 8,000 years ago, Grand Banks 1929, Papua New Guinea 1998 (Tappin, 2001). The Grand Banks and Papua New Guinea tsunamis came from earthquakes which destabilized sediments, causing them to flow into the ocean and generate a tsunami. They dissipated before traveling transoceanic distances.
The cause of the Storegga sediment failure is unknown. Possibilities include an overloading of the sediments, an earthquake or a release of gas hydrates (methane etc.)
The 1960 Valdivia earthquake (Mw 9.5) (19:11 hrs UTC), 1964 Alaska earthquake (Mw 9.2), 2004 Indian Ocean earthquake (Mw 9.2) (00:58:53 UTC) and 2011 Tōhoku earthquake (Mw9.0) are recent examples of powerful megathrust earthquakes that generated tsunamis (known as teletsunamis) that can cross entire oceans. Smaller (Mw 4.2) earthquakes in Japan can trigger tsunamis (called local and regional tsunamis) that can only devastate nearby coasts, but can do so in only a few minutes.
In the 1950s, it was discovered that larger tsunamis than had previously been believed possible could be caused by giant landslides. These phenomena rapidly displace large water volumes, as energy from falling debris or expansion transfers to the water at a rate faster than the water can absorb. Their existence was confirmed in 1958, when a giant landslide in Lituya Bay, Alaska, caused the highest wave ever recorded, which had a height of 524 metres (over 1700 feet). The wave didn't travel far, as it struck land almost immediately. Two people fishing in the bay were killed, but another boat amazingly managed to ride the wave. Scientists named these waves megatsunami.
Scientists discovered that extremely large landslides from volcanic island collapses can generate megatsunamis that can cross oceans.
Etymology and History of Tsunami
7:32 AM
Posted by Disaster
The term tsunami comes from the Japanese 津波, composed of the two kanji 津 (tsu) meaning "harbor" and 波 (nami), meaning "wave". (For the plural, one can either follow ordinary English practice and add an s, or use an invariable plural as in the Japanese.)
Tsunami are sometimes referred to as tidal waves. In recent years, this term has fallen out of favor, especially in the scientific community, because tsunami actually have nothing to do with tides. The once-popular term derives from their most common appearance, which is that of an extraordinarily high tidal bore. Tsunami and tides both produce waves of water that move inland, but in the case of tsunami the inland movement of water is much greater and lasts for a longer period, giving the impression of an incredibly high tide. Although the meanings of "tidal" include "resembling" or "having the form or character of" the tides, and the term tsunami is no more accurate because tsunami are not limited to harbours, use of the term tidal wave is discouraged by geologists and oceanographers.
There are only a few other languages that have an equivalent native word. In the Tamil language, the word is aazhi peralai. In the Acehnese language, it is ië beuna or alôn buluëk (Depending on the dialect. Note that in the fellow Austronesian language of Tagalog, a major language in the Philippines, alon means "wave".) On Simeulue island, off the western coast of Sumatra in Indonesia, in the Defayan language the word is smong, while in the Sigulai language it is emong.
As early as 426 B.C. the Greek historian Thucydides inquired in his book History of the Peloponnesian War about the causes of tsunami, and was the first to argue that ocean earthquakes must be the cause.
The cause, in my opinion, of this phenomenon must be sought in the earthquake. At the point where its shock has been the most violent the sea is driven back, and suddenly recoiling with redoubled force, causes the inundation. Without an earthquake I do not see how such an accident could happen.
The Roman historian Ammianus Marcellinus (Res Gestae 26.10.15-19) described the typical sequence of a tsunami, including an incipient earthquake, the sudden retreat of the sea and a following gigantic wave, after the 365 A.D. tsunami devastated Alexandria.
While Japan may have the longest recorded history of tsunamis, the sheer destruction caused by the 2004 earthquake and tsunami event mark it as the most devastating of its kind in modern times, killing around 230,000 people. The Sumatran region is not unused to tsunamis either, with earthquakes of varying magnitudes regularly occurring off the coast of the island.Tsunami
7:30 AM
Posted by Disaster
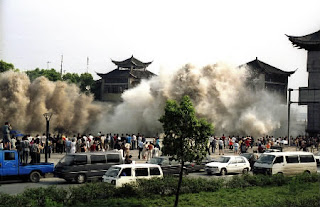
A tsunami also called a tsunami wave train, and at one time incorrectly referred to as a tidal wave, is a series of water waves caused by the displacement of a large volume of a body of water, usually an ocean, though it can occur in large lakes. Tsunamis are a frequent occurrence in Japan; approximately 195 events have been recorded. Owing to the immense volumes of water and the high energy involved, tsunamis can devastate coastal regions.
Earthquakes, volcanic eruptions and other underwater explosions (including detonations of underwater nuclear devices), landslides glacier calvings and other mass movements, meteorite ocean impacts or similar impact events, and other disturbances above or below water all have the potential to generate a tsunami.
The Greek historian Thucydides was the first to relate tsunami to submarine earthquakes,[5][6] but the understanding of a tsunami's nature remained slim until the 20th century and is the subject of ongoing research. Many early geological, geographical, and oceanographic texts refer to tsunamis as "seismic sea waves."
Some meteorological conditions, such as deep depressions that cause tropical cyclones, can generate a storm surge, called a meteotsunami, which can raise tides several metres above normal levels. The displacement comes from low atmospheric pressure within the centre of the depression. As these storm surges reach shore, they may resemble (though are not) tsunamis, inundating vast areas of land.
Limnic Eruption Effect
7:12 AM
Posted by Disaster
Once an eruption occurs, a large CO2 cloud forms above the lake and expands to the neighbouring region. Because CO2 is denser than air, it has a tendency to sink to the ground while pushing breathable air up. As a result, life forms that need to breathe oxygen suffocate once the CO2 cloud reaches them, as there is very little oxygen in the cloud. The CO2 can make human bodily fluids very acidic, potentially causing CO2 poisoning. As victims gasp for air they actually hurt themselves more by sucking in the CO2 gas.
At Lake Nyos, the gas cloud descended from the lake into a nearby village where it settled, killing nearly everyone. In this eruption, some people as far as 25 km (15.5 miles) from the lake died. A change in skin color on some bodies led scientists to think that the gas cloud may have contained a dissolved acid such as hydrogen chloride as well, but that hypothesis is disputed. Many victims were found with blisters on their skin. This is believed to have been caused by pressure ulcers, which likely formed from the low levels of oxygen present in the blood of those asphyxiated by the carbon dioxide. Thousands of cattle and wild animals were also asphyxiated, but no official counts were made. On the other hand, vegetation nearby was mostly unaffected except for that which grew immediately adjacent to the lake. There the vegetation was damaged or destroyed by a 5-meter (16.4 ft.) tsunami from the violent eruption.
Limnic Eruption Causes
7:10 AM
Posted by Disaster
For a limnic eruption to occur, the lake must be nearly saturated with gas. In the two known cases, the major component was CO2, however, in Lake Kivu, scientists are concerned about the concentrations of methane gas as well. This CO2 may come from volcanic gas emitted from under the lake or from decomposition of organic material. Before a lake is saturated, it behaves like an unopened carbonated beverage (soft drink): the CO2 is dissolved in the water. In both the lake and the soft drink, CO2 dissolves much more readily at higher pressure. This is why bubbles in a can of soda only form after the drink is open; the pressure is released and the CO2 comes out of solution. In the case of lakes, the bottom is at a much higher pressure; the deeper it is, the higher the pressure at the bottom. This means that huge amounts of CO2 can be dissolved in large, deep lakes. Also, CO2 dissolves more readily in cooler water, such as that at the bottom of a lake. A small rise in water temperature can lead to the release of a large amount of CO2.
Once the lake is saturated with CO2, it is very unstable. A trigger is all that is needed to set off an eruption. In the case of the 1986 eruption at Lake Nyos, landslides were the suspected triggers, but an actual volcanic eruption, an earthquake, or even wind and rain storms are other possible triggers. In any case, the trigger pushes some of the saturated water higher in the lake, where the pressure is insufficient to keep the CO2 in solution. Bubbles start forming and the water is lifted even higher in the lake (buoyancy), where even more of the CO2 comes out of solution. This process forms a column of gas. At this point the water at the bottom of this column is pulled up by suction, and it too loses its CO2 in a runaway process. This eruption pours CO2 into the air and can also displace water to form a tsunami.
There are several reasons this type of eruption is very rare. First, there must be a source of the CO2, so only regions with volcanic activity are at risk. Second, temperate lakes, such as North America's Great Lakes, turn over each spring and fall as a result of seasonal air temperature changes, mixing water from the bottom and top of the lake, so CO2 that builds up at the bottom of the lake is brought to the top where the pressure is too low for it to stay in solution and it escapes into the atmosphere. Finally, a lake must be quite deep to have enough pressure to dissolve large volumes of CO2. So only in deep, stable, tropical, volcanic lakes such as Lake Nyos are limnic eruptions possible.
Limnic Eruption - Lake Overturn
7:07 AM
Posted by Disaster

A limnic eruption or lake overturn, is a rare type of natural disaster in which carbon dioxide (CO2) suddenly erupts from deep lake water, suffocating wildlife, livestock and humans. Such an eruption may also cause tsunamis in the lake as the rising CO2 displaces water. Scientists believe landslides, volcanic activity, or explosions can trigger such an eruption. Lakes in which such activity occurs may be known as limnically active lakes or exploding lakes. Some features of limnically active lakes include:
- CO2-saturated incoming water
- A cool lake bottom indicating an absence of direct volcanic interaction with lake waters
- An upper and lower thermal layer with differing CO2 saturations
- Proximity to areas with volcanic activity