Earthquake
An earthquake (also known as a quake, tremor or temblor) is the result of a sudden release of energy in the Earth's crust that creates seismic waves.

Tsunami
A tsunami also called a tsunami wave train, and at one time incorrectly referred to as a tidal wave, is a series of water waves caused by the displacement of a large volume of a body of water, usually an ocean, though it can occur in large lakes.

Tornado
A tornado (often referred to as a twister or, erroneously, a cyclone) is a violent, dangerous, rotating column of air that is in contact with both the surface of the earth and a cumulonimbus cloud or, in rare cases, the base of a cumulus cloud.

Floods
A flood is an overflow of an expanse of water that submerges land. The EU Floods directive defines a flood as a temporary covering by water of land not normally covered by water

Volcanic Eruptions
Volcanoes can cause widespread destruction and consequent disaster through several ways. The effects include the volcanic eruption itself that may cause harm following the explosion of the volcano or the fall of rock.

Large Igneous Provinces
7:47 AM
Posted by Disaster
Highly active periods of volcanism in what are called large igneous provinces have produced huge oceanic plateaus and flood basalts in the past. These can comprise hundreds of large eruptions, producing millions of cubic kilometers of lava in total. No large flood basalt type eruptions have occurred in human history, the most recent having occurred over 10 million years ago. They are often associated with breakup of supercontinents such as Pangea in the geologic record, and may have contributed to a number of mass extinctions. Most large igneous provinces have either not been studied thoroughly enough to establish the size of their component eruptions, or are not preserved well enough to make this possible. Many of the eruptions listed above thus come from just two large igneous provinces: the Paraná and Etendeka traps and the Columbia River Basalt Group. The latter is the most recent large igneous province, and also one of the smallest. A list of large igneous provinces follows to provide some indication of how many large eruptions may be missing from the lists given here.
Igneous province | Age (Ma) | Location | Volume (millions of km3) | Notes |
---|---|---|---|---|
Ontong Java–Manihiki–Hikurangi Plateau | 121 | Southwest Pacific Ocean | 59–77[n 5] | Largest igneous body on Earth, later split into three widely separated oceanic plateaus, with a fourth component perhaps now accreted onto South America. Possibly linked to the Louisville hotspot. |
Kerguelen Plateau–Broken Ridge | 112 | South Indian Ocean, Kerguelen Islands | 17[n 5] | Linked to the Kerguelen hotspot. Volume includes Broken Ridge and the Southern and Central Kerguelen Plateau (produced 120–95 Ma), but not the Northern Kerguelen Plateau (produced after 40 Ma). |
North Atlantic Igneous Province | 55.5 | North Atlantic Ocean | 6.6[n 6] | Linked to the Iceland hotspot. |
Mid-Tertiary ignimbrite flare-up | 32.5 | Southwest United States: mainly in Colorado, Nevada, Utah, and New Mexico | 5.5 | Mostly andesite to rhyolite explosive (.5 km3) to effusive (5 km3) eruptions, 25–40 Ma. Includes many volcanic centers, including the San Juan volcanic field. |
Caribbean large igneous province | 88 | Caribbean-Colombian oceanic plateau | 4 | Linked to the Galápagos hotspot. |
Siberian Traps | 249.4 | Siberia, Russia | 1–4 | Possibly the largest outpouring of lava on land ever recorded, thought to have caused Permian-Triassic extinction, largest mass extinction event ever. |
Karoo-Ferrar | 183 | Southern Africa, Antarctica | 2.5 | Formed as Gondwana broke up |
Paraná and Etendeka traps | 133 | Brazil/Angola and Namibia | 2.3 | Linked to the Tristan hotspot |
Central Atlantic Magmatic Province | 200 | Laurasia continents | 2 | Formed as Pangea broke up |
Deccan Traps | 65.5 | Deccan Plateau, India | 1.5 | May have helped kill the dinosaurs. |
Emeishan Traps | 256.5 | Southwestern China | 1 | Along with Siberian Traps, may have contributed to the Permian–Triassic extinction event. |
Coppermine River Group | 1267 | Mackenzie Large Igneous Province/Canadian Shield | 0.65 | Consists of at least 150 individual flows. |
Afro-Arabian flood volcanism | 28.5 | Ethiopia/Yemen/Afar, Arabian-Nubian Shield | 0.35 | Associated with silicic, explosive tuffs |
Columbia River Basalt Group | 16 | Pacific Northwest, United States | 0.18 | Well exposed by Missoula Floods in the Channeled Scablands. |
Largest Volcanic Effusive Eruptions
7:45 AM
Posted by Disaster
Effusive eruptions involve a relatively gentle, steady outpouring of lava rather than large explosions. They can continue for years or decades, producing extensive fluid mafic lava flows. For example, Kīlauea on Hawaiʻi has continued erupting from 1983 to the present, producing 2.7 km3 (1 cu mi) of lava covering more than 100 km2 (40 sq mi). The largest effusive eruption in history occurred in Iceland during the 1783–1784 eruption of Laki, which produced about 15 km3 (4 cu mi) of lava and killed one fifth of Iceland's population. The ensuing disruptions to the climate may also have killed millions elsewhere.
Eruption | Age (Ma) | Location | Volume (km3) | Notes |
---|---|---|---|---|
Mahabaleshwar–Rajahmundry Traps (Upper) | 64.8 | Deccan traps, India | 9,300 | |
Wapshilla Ridge flows | ~15.5 | Columbia River Basalt Group, United States | 5,000–10,000 | Member comprises 8–10 flows with a total volume of ~50,000 km3 |
McCoy Canyon flow | 15.6 | Columbia River Basalt Group, United States | 4,300 | |
Umtanum flows | ~15.6 | Columbia River Basalt Group, United States | 2,750 | Two flows with a total volume of 5,500 km3 |
Sand Hollow flow | 15.3 | Columbia River Basalt Group, United States | 2,660 | |
Pruitt Draw flow | 16.5 | Columbia River Basalt Group, United States | 2,350 | |
Museum flow | 15.6 | Columbia River Basalt Group, United States | 2,350 | |
Moonaree Dacite | 1591 | Gawler Range Volcanics, Australia | 2,050 | One of the oldest large eruptions preserved |
Rosalia flow | 14.5 | Columbia River Basalt Group, United States | 1,900 | |
Joseph Creek flow | 16.5 | Columbia River Basalt Group, United States | 1,850 | |
Ginkgo Basalt | 15.3 | Columbia River Basalt Group, United States | 1,600 | |
California Creek—Airway Heights flow | 15.6 | Columbia River Basalt Group, United States | 1,500 | |
Stember Creek flow | 15.6 | Columbia River Basalt Group, United States | 1,200 |
Largest Volcanic Explosive Eruptions
7:41 AM
Posted by Disaster
In explosive eruptions, the eruption of magma is driven by the rapid release of pressure, often involving the explosion of gas previously dissolved within the material. The most famous and destructive historical eruptions are mainly of this type. An eruptive phase can consist of a single eruption, or a sequence of several eruptions spread over several days, weeks or months. Explosive eruptions usually involve thick, highly viscous felsic magma, high in volatiles like water vapor and carbon dioxide. Pyroclastic materials are the primary product, typically in the form of tuff. Eruptions the size of that at Lake Toba 74 thousand years ago (2800 km3 or more) occur worldwide every 50,000 to 100,000 years.
Volcano—Eruption | Age (Ma) | Location | Volume (km3) | Notes |
---|---|---|---|---|
Guarapuava —Tamarana—Sarusas | 132 | Paraná and Etendeka traps | 8,600 | |
Santa Maria—Fria | ~132 | Paraná and Etendeka traps | 7,800 | |
Guarapuava —Ventura | ~132 | Paraná and Etendeka traps | 7,600 | |
Sam Ignimbrite and Green Tuff | 29.5 | Yemen | 6,800 | Volume includes 5550 km³ of distal tuffs. This estimate is uncertain to a factor of 2 or 3. |
Goboboseb–Messum volcanic centre—Springbok quartz latite unit | 132 | Paraná and Etendeka traps, Brazil and Namibia | 6,340 | |
Caxias do Sul—Grootberg | ~132 | Paraná and Etendeka traps | 5,650 | |
La Garita Caldera—Fish Canyon tuff | 27.8 | San Juan volcanic field, Colorado | 5,000 | Commonly regarded as the largest tuff ever measured on Earth, or largest confidently-measured tuff on earth. It is part of at least 20 large caldera-forming eruptions in the San Juan volcanic field and surrounding area that formed around 26 to 35 Ma. |
Jacui—Goboboseb II | ~132 | Paraná and Etendeka traps | 4,350 | |
Ourinhos—Khoraseb | ~132 | Paraná and Etendeka traps | 3,900 | |
Jabal Kura'a Ignimbrite | 29.6 | Yemen | 3,800 | Volume estimate is uncertain to a factor of 2 or 3. |
Windows Butte tuff | 31.4 | William's Ridge, central Nevada | 3,500 | Part of the Mid-Tertiary ignimbrite flare-up |
Anita Garibaldi—Beacon | ~132 | Paraná and Etendeka traps | 3,450 | |
Indian Peak Caldera Complex—Wah Wah Springs tuff | 29.5 | Eastern Nevada/Western Utah | 3,200 | Indian Peak Caldera Complex total volume over 10,000 cubic km, Wah Wah Springs tuff being the largest |
Oxaya ignimbrites | 19 | Chile | 3,000 | Really a regional correlation of many ignimbrites originally thought to be distinct |
Lund Tuff | 29 | Great Basin, USA | 3,000 | Similar in composition to the Fish Canyon Tuff |
Lake Toba—Youngest Toba Tuff | 0.073 | Sunda Arc, Indonesia | 2,800 | Largest eruption on earth in at least the last 25 million years, responsible for the Toba catastrophe theory, a population bottleneck of the human species |
Pacana Caldera—Atana ignimbrite | 4 | Chile | 2,800 | Forms a resurgent caldera. |
Iftar Alkalb—Tephra 4 W | 29.5 | Afro-Arabian | 2,700 | |
Yellowstone caldera—Huckleberry Ridge Tuff | 2.059 | Yellowstone hotspot | 2,450 | Largest Yellowstone eruption on record |
Whakamaru | 0.254 | Taupo Volcanic Zone, New Zealand | 2,000 | Largest in the Southern Hemisphere in the Late Quaternary |
Palmas BRA-21—Wereldsend | 29.5 | Paraná and Etendeka traps | 1,900 | |
Kilgore tuff | 4.3 | Near Kilgore, Idaho | 1,800 | Last of the eruptions from the Heise volcanic field |
Sana'a Ignimbrite—Tephra 2W63 | 29.5 | Afro-Arabian | 1,600 | |
Millbrig eruptions—Bentonites | 454 | England, exposed in Northern Europe and Eastern US | 1,509 | One of the oldest large eruptions preserved |
Blacktail tuff | 6.5 | Blacktail, Idaho | 1,500 | First of several eruptions from the Heise volcanic field |
Emory Caldera—Kneeling Nun tuff | 33 | Southwestern New Mexico | 1,310 | |
Timber Mountain tuff | 11.6 | Southwestern Nevada | 1,200 | Also includes a 900 cubic km tuff as a second member in the tuff |
Paintbrush tuff (Topopah Spring Member) | 12.8 | Southwestern Nevada | 1,200 | Related to a 1000 cubic km tuff (Tiva Canyon Member) as another member in the Paintbrush tuff |
Bachelor—Carpenter Ridge tuff | 28 | San Juan volcanic field | 1,200 | Part of at least 20 large caldera-forming eruptions, including the world's largest, the Fish Canyon tuff in the San Juan volcanic field and surrounding area that formed around 26 to 35 Ma |
Bursum—Apache Springs Tuff | 28.5 | Southern New Mexico | 1,200 | Related to a 1050 cubic km tuff, the Bloodgood Canyon tuff |
Taupo Volcano—Oruanui eruption | 0.027 | Taupo volcanic zone, New Zealand | 1,170 | Most recent VEI 8 eruption |
Huaylillas Ignimbrite | 15 | Bolivia | 1,100 | Predates half of the uplift of the central Andes |
Bursum—Bloodgood Canyon tuff | 28.5 | Southern New Mexico | 1,050 | Related to a 1200 cubic km tuff, the Apache Springs tuff |
Yellowstone Caldera—Lava Creek Tuff | 0.639 | Yellowstone hotspot | 1,000 | Last large eruption in the Yellowstone National Park area |
Cerro Galán | 2.2 | Catamarca Province, Argentina | 1,000 | Elliptical caldera is ~35 km wide |
Paintbrush tuff (Tiva Canyon Member) | 12.7 | Southwestern Nevada | 1,000 | Related to a 1200 cubic km tuff (Topopah Spring Member) as another member in the Paintbrush tuff |
San Juan—Sapinero Mesa Tuff | 28 | San Juan volcanic field | 1,000 | Part of at least 20 large caldera-forming eruptions, including the world's largest, the Fish Canyon tuff in the San Juan volcanic field and surrounding area that formed around 26 to 35 Ma |
Uncompahgre—Dillon & Sapinero Mesa Tuffs | 28.1 | San Juan volcanic field | 1,000 | Part of at least 20 large caldera-forming eruptions, including the world's largest, the Fish Canyon tuff in the San Juan volcanic field and surrounding area that formed around 26 to 35 Ma |
Platoro—Chiquito Peak tuff | 28.2 | San Juan volcanic field | 1,000 | Part of at least 20 large caldera-forming eruptions, including the world's largest, the Fish Canyon tuff in the San Juan volcanic field and surrounding area that formed around 26 to 35 Ma |
Mount Princeton—Wall Mountain tuff | 35.3 | Thirtynine Mile volcanic area, Colorado | 1,000 | Helped cause the exceptional preservation at Florissant Fossil Beds National Monument |
Subglacial Eruption
7:14 AM
Posted by Disaster

Subglacial eruption: 1 water vapor cloud, 2 lake, 3 ice, 4 layers of lava and ash, 5 strata, 6 pillow lava, 7 magma conduit, 8 magma chamber, 9 dike.
A subglacial eruption is a volcanic eruption that has occurred under ice, or under a glacier. Subglacial eruptions can cause dangerous floods, lahars and create hyaloclastite and pillow lava. Subglacial eruptions sometimes form a subglacial volcano called a tuya. Tuyas in Iceland are called table mountains because of their flat tops. Tuya Butte, in northern British Columbia is an example of a tuya. A tuya may be recognized by its stratigraphy, which typically consists of a basal layer of pillow basalts overlain by hyaloclastite breccia, tuff, and capped off by a lava flow. The pillow lavas formed first as a result of subaqueous eruptions in glacial meltwater. Once the vent reaches shallower water, eruptions become phreatomagmatic, depositing the hyaloclastite breccia. Once the volcano emerges through the ice, it erupts lava, forming the flat capping layer of a tuya.
The thermodynamics of subglacial eruptions are very poorly understood. Rare published studies indicate that plenty of heat is contained in the erupted lava, with 1 unit-volume of magma sufficient to melt about 10 units of ice. However, the rapidity by which ice is melted is unexplained, and in real eruptions the rate is at least an order of magnitude faster than existing predictions.
Antarctica eruption
On January, 2008, the British Antarctic Survey (Bas) scientists led by Hugh Corr and David Vaughan, reported (in the journal Nature Geoscience) that 2,200 years ago, a volcano erupted under the Antarctica ice sheet (based on airborne survey with radar images). The biggest eruption in the last 10,000 years, the volcanic ash was found deposited on the ice surface under the Hudson Mountains, close to Pine Island Glacier. The ash covered an area the size of New Hampshire and was probably deposited from a 12 km high ash plume. Researchers have detected a mountainous peak some 100 meters beneath the surface believed to be the top of the tuya associated with this eruption.
List of volcanoes with Holocene subglacial eruptions
Iceland
- Bárðarbunga
- Eyjafjallajökull
- Grímsvötn
- Hveravellir
- Katla
- Kverkfjöll
- Prestahnúkur
- Snæfellsjökull
- Torfajökull
- Öræfajökull
South America
- Lautaro
- Sollipulli
Submarine Eruption
7:08 AM
Posted by Disaster

Diagram of a Submarine eruption: 1. Water vapor cloud 2. Water 3. Stratum 4. Lava flow 5. Magma conduit 6. Magma chamber 7. Dike 8. Pillow lava.
Submarine eruptions are a type of volcanic eruption that occurs underwater. An estimated 75% of the total volcanic eruptive volume is generated by submarine eruptions near mid ocean ridges alone, however because of the problems associated with detecting deep sea volcanics, they remained virtually unknown until advances in the 1990s made it possible to observe them.
Submarine eruptions are generated by seamounts (underwater volcanoes), and are driven by one of two processes. Volcanoes near plate boundaries and mid-ocean ridges are built by the decompression melting of mantle rock that floats up to the crustal surface. Eruptions near subducting zones, meanwhile, are driven by subducting plates that adds volatiles to the rising plate, raising its melting point. Each process generates different rock; mid-ocean ridge volcanics are primarily basaltic, whereas subduction flows are mostly calc-alkaline, and more explosive and viscous.
Spreading rates along mid-ocean ridges vary widely, from 2 cm (0.8 in) per year at the Mid-Atlantic Ridge, to up to 16 cm (6 in) along the East Pacific Rise. Higher spreading rates are a probably cause for higher levels of volcanism. The technology for studying seamount eruptions did not exist until advancements in hydrophone technology made it possible to "listen" to acoustic waves, known as T-waves, released by submarine earthquakes associated with submarine volcanic eruptions. The reason for this is that land-based seismometers cannot detect sea-based earthquakes below a magnitude of 4, but acoustic waves travel well in water and long periods of time. A system in the North Pacific, maintained by the United States Navy and originally intended for the detection of submarines, has detected an event on average every 2 to 3 years.
The most common underwater flow is pillow lava, a circular lava flow named after its unusual shape. Less common are glassy, marginal sheet flows, indicative of larger-scale flows. Volcaniclastic sedimentary rocks are common in shallow-water environments. As plate movement starts to carry the volcanoes away from their eruptive source, eruption rates start to die down, and water erosion grinds the volcano down. The final stages of eruption caps the seamount in alkalic flows. There are about 100,000 deepwater volcanoes in the world, although most are beyond the active stage of their life. Some exemplery seamounts are Loihi Seamount, Bowie Seamount, Cross Seamount, and Denson Seamount.
Surtseyan Eruption
7:05 AM
Posted by Disaster

Surtseyan eruption: 1 water vapor cloud, 2 cupressoid ash, 3 crater, 4 water, 5 layers of lava and ash, 6 stratum, 7 magma conduit, 8 magma chamber, 9 dike.
A Surtseyan eruption is a type of volcanic eruption that takes place in shallow seas or lakes. It is named after the island of Surtsey off the southern coast of Iceland.
These eruptions are commonly phreatomagmatic eruptions, representing violent explosions caused by rising basaltic or andesitic magma coming into contact with abundant, shallow groundwater or surface water. Tuff rings, pyroclastic cones of primarily ash, are built by explosive disruption of rapidly cooled magma. Other examples of these volcanoes: Capelinhos, Faial Island, Azores; and Taal Volcano, Batangas, Philippines.
Characteristics of Surtseyan Eruption
Although similar in nature to phreatomagmatic eruptions, there are several specific characteristics:
- Physical nature of magma: viscous; basaltic.
- Character of explosive activity: violent ejection of solid, warm fragments of new magma; continuous or rhythmic explosions; base surges.
- Nature of effusive activity: short, locally pillowed, lava flows; lavas may be rare.
- Nature of dominant ejecta: lithic, blocks and ash; often accretionary lapilli; spatter, fusiform bombs and lapilli absent.
- Structures built around vent: tuff rings
Plinian Eruption
6:59 AM
Posted by Disaster
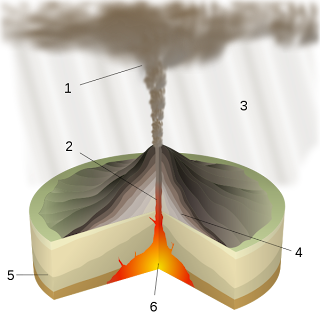
Diagram of a Plinian eruption: 1. Ash plume 2. Magma conduit 3. Volcanic ash rain 4. Layers of lava and ash 5. Stratum 6. Magma chamber.
Plinian eruptions, also known as 'Vesuvian eruptions', are volcanic eruptions marked by their similarity to the eruption of Mount Vesuvius in AD 79 (as described in a letter written by Pliny the Younger, and which killed his uncle Pliny the Elder).
Plinian eruptions are marked by columns of gas and volcanic ash extending high into the stratosphere, a high layer of the atmosphere. The key characteristics are ejection of large amount of pumice and very powerful continuous gas blast eruptions.
Short eruptions can end in less than a day, but longer events can take several days to months. The longer eruptions begin with production of clouds of volcanic ash, sometimes with pyroclastic flows. The amount of magma erupted can be so large that the top of the volcano may collapse, resulting in a caldera. Fine ash can deposit over large areas. Plinian eruptions are often accompanied by loud noises, such as those generated by Krakatoa.
The lava is usually rhyolitic and rich in silicates. Basaltic lavas are unusual for Plinian eruptions; the most recent example is the 1886 eruption of Mount Tarawera.Pliny's description
Pliny described his uncle's involvement from the first observation of the eruption:
On the 24th of August, about one in the afternoon, my mother desired him to observe a cloud which appeared of a very unusual size and shape. He had just taken a turn in the sun and, after bathing himself in cold water, and making a light luncheon, gone back to his books: he immediately arose and went out upon a rising ground from whence he might get a better sight of this very uncommon appearance. A cloud, from which mountain was uncertain, at this distance (but it was found afterwards to come from Mount Vesuvius), was ascending, the appearance of which I cannot give you a more exact description of than by likening it to that of a pine tree, for it shot up to a great height in the form of a very tall trunk, which spread itself out at the top into a sort of branches; occasioned, I imagine, either by a sudden gust of air that impelled it, the force of which decreased as it advanced upwards, or the cloud itself being pressed back again by its own weight, expanded in the manner I have mentioned; it appeared sometimes bright and sometimes dark and spotted, according as it was either more or less impregnated with earth and cinders. This phenomenon seemed to a man of such learning and research as my uncle extraordinary and worth further looking into.
– Sixth Book of Letters, Letter 16
Pliny the Elder set out to rescue the victims from their perilous position on the shore of the Bay of Naples, and launched his galleys, crossing the bay to Stabiae (near the modern town of Castellammare di Stabia). Pliny the Younger provided an account of his death, and suggested that he collapsed and died through inhaling poisonous gases emitted from the volcano. His body was found interred under the ashes of the Vesuvius with no apparent injuries on 26 August, after the plume had dispersed, confirming asphyxiation or poisoning.
Ultra Plinian
According to the Smithsonian Institution's Volcanic Explosivity Index, a VEI of 6 to 8 is classified as "Ultra Plinian." They are defined by ash plumes over 25 km (16 mi) high and a volume of erupted material 10 km3 (2 cu mi) to 1,000 km3 (200 cu mi) in size. Eruptions in the "Ultra Plinian" category include Lake Toba (74 ka), Tambora (1815), and Krakatoa (1883).
Examples of Plinian Eruption
- The 2010 eruptions of Eyjafjallajökull in Iceland
- The June 2009 eruption of Sarychev Peak in Russia
- The 1991 Mount Pinatubo eruption in Luzon in the Philippines;
- The 1980 eruption of Mount St. Helens in USA
- The 1883 eruption of Krakatoa in Indonesia
- The 1815 eruption of Mount Tambora in Indonesia
- The 1667 and 1739 eruptions of Mount Tarumae in Japan
- The AD 79 eruption of Mount Vesuvius in Italy, which was the prototypical Plinian eruption.
- The 1645 BC eruption of Santorini in Greece
- The 4860 BC eruption forming Crater Lake in USA
- The Long Valley Caldera eruption in USA over 760,000 years ago.

Peléan Eruption
6:56 AM
Posted by Disaster

Peléan eruption: 1 Ash plume, 2 Volcanic ash rain, 3 Lava dome, 4 Volcanic bomb, 5 Pyroclastic flow, 6 Layers of lava and ash, 7 Strata, 8 Magma conduit, 9 Magma chamber, 10 Dike
Peléan eruptions are a type of volcanic eruption. They can occur when viscous magma, typically of rhyolitic or andesitic type, is involved, and share some similarities with Vulcanian eruptions. The most important characteristics of a Peléan eruption is the presence of a glowing avalanche of hot volcanic ash, a pyroclastic flow. Formation of lava domes is another characteristical feature. Short flows of ash or creation of pumice cones may be observed as well.
The initial phases of eruption are characterized by pyroclastic flows. The tephra deposits have lower volume and range than the corresponding Plinian and Vulcanian eruptions. The viscous magma then forms a steep-sided dome or volcanic spine in the volcano's vent. The dome may later collapse, resulting in flows of ash and hot blocks. The eruption cycle is usually completed in few years, but in some cases may continue for decades, like in the case of Santiaguito.
The 1902 explosion of Mount Pelée is the first described case of a Peléan eruption, and gave it its name.
Some other examples include the following:
- the 1948-1951 eruption of Hibok-Hibok;
- the 1951 eruption of Mount Lamington, which remains the most detailed observation of this kind;
- the 1956 eruption of Bezymianny;
- the 1968 eruption of Mayon Volcano;
- and the 1980 eruption of Mount St. Helens.
Vulcanian Eruption
6:52 AM
Posted by Disaster

The term Vulcanian was first used by Giuseppe Mercalli, witnessing the 1888-1890 eruptions on the island of Vulcano. His description of the eruption style is now used all over the world for eruptions characterised by a dense cloud of ash-laden gas exploding from the crater and rising high above the peak. Mercalli described vulcanian eruptions as "...Explosions like cannon fire at irregular intervals..." Their explosive nature is due to increased silica content of the magma. Almost all types of magma can be involved, but magma with about 55% or more silica (basalt–andesite) is most common. Increasing silica levels increase the viscosity of the magma which means increased explosiveness. They usually commence with phreatomagmatic eruptions which can be extremely noisy due the rising magma heating water in the ground. This is usually followed by the explosive clearing of the vent and the eruption column is dirty grey to black as old weathered rocks are blasted out of the vent. As the vent clears, further ash clouds become grey-white and creamy in colour, with convolutions of the ash similar to those of plinian eruptions.
Characteristics
Vulcanian eruptions display several common characteristics. The mass of rock ejected during the eruption is usually between 102 - 106 tonnes and contains a high proportion of non-juvinial material (> 50%). During active periods of volcanic activity, intervals between explosions vary from less than 1 minute (e.g. Anak Krakatoa) to about a day. Pyroclastic flows are also common features of this type of eruption. The gas streaming phase of these eruptions are characterised discrete canon-like explosions, which are a particular features of vulcanian eruptions. These expulsions of gas can reach supersonic velocities resulting in shock waves.
The tephra is dispersed over a wider areas than that from Strombolian eruptions. The pyroclastic rock and the base surge deposits form an ash volcanic cone, while the ash covers a large surrounding area. The eruption ends with a flow of viscous lava. Vulcanian eruptions may throw large metre-size blocks several hundred metres, occasionally up to several kilometres.
Vulcanian eruptions are dangerous to persons within several hundred metres of the vent. One feature of this type of eruption is the "Volcanic bomb." These can be blocks often 2 to 3 m in dimensions. At Galeras a vulcanian eruption ejected bombs which impacted with several volcanologists who were in the crater and many died or suffered terrible injuries.
1930 Eruption of Stromboli
The 11 September 1930 eruption of Stromboli was a vulcanian eruption. It started at 08:10 hours (local), when ash was vented for about 10 minutes. Then at 09:52 two incredibly powerful explosions occurred which shook the whole island. Blocks were hurled about 2 km. These fell out of the sky smashing through buildings etc. A tsunami 2 to 2.5m high was generated. By 10:40 the explosive phase of the eruption was over. Expulsion of lava followed, this flowed down the Sciara del Fuoco, lasting into the night. At the same time incandescent scoria flowed down the Vallonazzo Valley and entered the sea near Piscità.
By the end, 6 people had died. Four fishermen died at sea when the avalanches of hot scoria caused the sea to become very disturbed. One person was killed in Stromboli village by falling blocks, and the 6th was killed by the tsunami. It is believed that water entered the conduit due to a partial collapse of the conduit. The water flashed into steam and took the easiest "escape route," via the open conduit. As it expanded in the molten magma it generated the two very large explosions.
Strombolian Eruption
6:48 AM
Posted by Disaster

Diagram of a Strombolian eruption: 1. Ash plume 2. Lapilli 3. Volcanic ash rain 4. Lava fountain 5. Volcanic bomb 6. Lava flow 7. Layers of lava and ash 8. Stratum 9. Dike 10. Magma conduit 11. Magma chamber 12. Sill
Strombolian eruptions are relatively low-level volcanic eruptions, named after the Italian volcano Stromboli, where such eruptions consist of ejection of incandescent cinder, lapilli and lava bombs to altitudes of tens to hundreds of meters. They are small to medium in volume, with sporadic violence.
They are defined as "...Mildly explosive at discrete but fairly regular intervals of seconds to minutes..."
The tephra typically glows red when leaving the vent, but its surface cools and assumes a dark to black color and may significantly solidify before impact. The tephra accumulates in the vicinity of the vent, forming a cinder cone. Cinder is the most common product, the amount of volcanic ash is typically rather minor.
The lava flows are more viscous, and therefore shorter and thicker, than the corresponding Hawaiian eruptions; it may or may not be accompanied by production of pyroclastic rock.
Instead the gas coalesces into bubbles, called gas slugs, that grow large enough to rise through the magma column, bursting near the top due to the decrease in pressure and throwing magma into the air. Each episode thus releases volcanic gases, sometimes as frequently as a few minutes apart. Gas slugs can form as deep as 3 kilometers, making them difficult to predict.
Strombolian eruptive activity can be very long-lasting because the conduit system is not strongly affected by the eruptive activity, so that the eruptive system can repeatedly reset itself. For example, the Parícutin volcano erupted continuously between 1943-1952, Mount Erebus, Antarctica has produced Strombolian eruptions for at least many decades, and Stromboli itself has been producing Strombolian eruptions for several thousand years.
Hawaiian Eruption
6:41 AM
Posted by Disaster

Hawaiian eruption: 1: Ash plume, 2: Lava fountain, 3: Crater, 4: Lava lake, 5: Fumaroles, 6: Lava flow, 7 Layers of lava and ash, 8: Stratum, 9: Sill, 10: Magma conduit, 11: Magma chamber, 12: Dike
A Hawaiian eruption is a type of volcanic eruption where lava flows from the vent in a relative gentle, low level eruption, so called because it is characteristic of Hawaiian volcanoes. Typically they are effusive eruptions, with basaltic magmas of low viscosity, low content of gases, and high temperature at the vent. Very little amount of volcanic ash is produced. This type of eruption occurs most often at hotspot volcanoes such as Kīlauea, though it can occur near subduction zones (e.g. Medicine Lake Volcano in California, United States) and rift zones. Another example of Hawaiian eruptions occurred on Surtsey from 1964 to 1967, when molten lava flowed from the crater to the sea.
Hawaiian eruptions may occur along fissure vents, such as during the eruption of Mauna Loa Volcano in 1950, or at a central vent, such as during the 1959 eruption in Kīlauea Iki Crater, which created a lava fountain 580 meters (1,900 ft) high and formed a 38 meter cone named Puʻu Puaʻi. In fissure-type eruptions, lava spurts from a fissure on the volcano's rift zone and feeds lava streams that flow downslope. In central-vent eruptions, a fountain of lava can spurt to a height of 300 meters or more (heights of 1600 meters were reported for the 1986 eruption of Mount Mihara on Izu Ōshima, Japan).
Hawaiian eruptions usually start by formation of a crack in the ground from which a curtain of incandescent magma or several closely spaced magma fountains appear. The lava can overflow the fissure and form ʻaʻā or pāhoehoe style of flows. When such an eruption from a central cone is protracted, it can form lightly sloped shield volcanoes, for example Mauna Loa or Skjaldbreiður in Iceland.
Petrology of Hawaiian Basalts
The key factors in generating a Hawaiian eruption are basaltic magma and a low percentage of dissolved water (less than one percent). The lower the water content, the more peaceful is the resulting flow. Almost all lava that comes from Hawaiian volcanoes is basalt in composition. Hawaiian basalts that make up almost all of the islands are tholeiite. These rocks are similar but not identical to those that are produced at ocean ridges. Basalt relatively richer in sodium and potassium (more alkaline) has erupted at the undersea volcano of Lōʻihi at the extreme southeastern end of the volcanic chain, and these rocks may be typical of early stages in the "evolution" of all Hawaiian islands. In the late stages of eruption of individual volcanoes, more alkaline basalt also was erupted, and in the very late stages after a period of erosion, rocks of unusual composition such as nephelinite were produced in very small amounts. These variations in magma composition have been investigated in great detail, in part to try to understand how mantle plumes may work.
Safety
Hawaiian eruptions are usually the most attractive to tourists and are the safest because there is little danger from ash. However, Hawaiian eruptions are not always safe.
In 1790, 80 warriors marching on Kīlauea were killed in an eruption. On May 18, 1924, a plantation accountant named Truman Taylor who was sightseeing on Kīlauea's caldera, was hit with debris from an explosion. Although rushed to hospital, Taylor succumbed to his injuries later that day.